“There’s Nothing Like an Observation that Confirms Einstein’s Ideas!”
- Interview with Barry Barish
- 2 févr. 2018
- 10 min de lecture
Barry Barish played a key role in detecting gravitational waves, mobilising the scientific community in the international Ligo project, which first observed the collision of two black holes in 2015. The American physicist talks about one of the greatest collaborations in contemporary science.
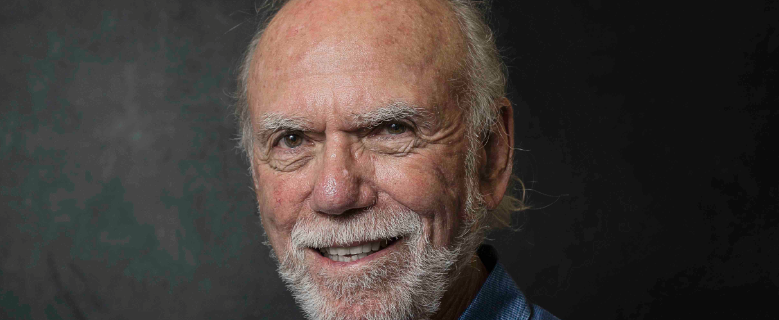
Photo crédit © Caltech
He doesn’t believe it at first, on 14th September 2015, when he sees the wild spikes captured by the instruments in the international Ligo project. The collision of two black holes jumps out to expert eyes. But Barry Barish is a prudent man. His experience tells him that the stakes are too high to risk precipitating the announcement; this is about completing the quest of a century, since Einstein’s prediction of the existence of gravitational waves, and opening up a new era in astronomy, because there is much to learn from the collisions of black holes and other strange stars. Once checked, there is no longer any doubt. The event has a historical feel to it. This was felt by many of the thousands of members of the Ligo and Virgo (for data analysis) projects. From 1994 to 2005, Barry Barish kept Ligo afloat despite the failing project’s internal problems. Today he is just an ordinary member again, but he remains a privileged witness to this extraordinary scientific adventure that won him the Nobel Prize for Physics.
La Recherche Two years ago, gravitational waves emitted by the collision of two black holes were first detected by the Ligo instruments, and recently the fusion of two neutron stars was first observed, using the same waves. Finally, you shared the Nobel Prize for Physics with Rainer Weiss and Kip Thorne, also original founders of Ligo. Which event marked you the most?
Barry Barish All these events were exciting in their own way. When we first detected two black holes colliding, we worked hard for more than a month to convince ourselves that what we were seeing was real and not just an accident. There’s nothing like an observation that confirms Einstein’s ideas! Black holes are unique objects for accurately testing the theory of general relativity. As for the fusion of two neutron stars, it also emits electromagnetic waves - i.e. light - that other telescopes captured. This is all the more exciting for astrophysicists as neutron stars remain a mystery. These first observations are almost equivalent to the first observation of the sky through Galileo’s telescope four hundred years ago. That started a science that continues to this day. Similarly, direct detection of gravitational waves marks the start of a new type of astronomy. Personally for me, the highlight was the seminar I gave at CERN, near Geneva on 11th February 2016, the day we officially announced the first collision of black holes. I will never forget the excitement of my scientist colleagues.
How did the quest for gravitational waves happen?
It all started in 1916. Einstein published his General Theory of Relativity. He saw that his equations, describing gravity, shared some characteristics with electromagnetic equations, which described light waves, among other emissions. By following through with this analogy, he proposed the existence of gravitational waves. They are deformations in the fabric of space-time itself, caused by massive, accelerating objects. Of course, he never imagined it would one day be proven by experiment. Black holes and neutron stars were unknown objects at the time, although the theoretical existence of black holes was predicted in 1916 by the German astrophysicist Karl Schwarzschild.
It was still a while before these gravitational waves became of interest to physicists...
Yes, many theorists still had doubts, including Einstein himself. In 1957, a congress was organised on the subject in Chapel Hill in North Carolina. The British physicist Felix Pirani proposed a convincing theoretical derivation for the existence of these waves, and his American counterpart, Richard Feynman, showed that they could carry energy. So, they have a physical effect. Then it was the right time to conduct experiments to try and detect them. The first to do so was Joseph Weber. He made some aluminium bars, two metres by one metre, that were supposed to vibrate when a gravitational wave passed through them. It was a clever idea, except he took “noise” for a signal and declared it to be a gravitational wave. Other experiments proved him wrong, but unfortunately, he never accepted it. One of his students on the other hand, Robert Forward, took an idea already put forward by the Soviet physicists Mikhail Gertsenshtein and Vladislav Pustovoit in 1962: the idea of using interferometers to detect gravitational waves.
What is an interferometer?
This is an instrument in which a beam of light is split into two parts, that run different paths before being sent back by a mirror and recombined. Measuring the recombined signal determines if there was a variation in the relative length of the two paths, which is the case if a gravitational wave passes through the interferometer. The longer the paths, the easier it is to detect small variations. Rainer Weiss was one of the first people to design what a massive interferometer would look like. There were several tests in the 1980s; an instrument, 30 metres long in Garching, Germany, and another 40 metres long at the California Institute of Technology (Caltech).
Were these long enough to have hope of detecting gravitational waves?
No! Instead of 40 metres, we needed 4 kilometres. There is an intuition that guides testers in evaluating the viability of a project; increasing the performance of an instrument by a factor of 2 is not usually a problem, but increasing it by a factor of 10 is a dream. In this case, we needed to build an instrument 100 times larger! In addition, one of the major difficulties lies in the fact that the instrument, and especially the mirrors, need to be insulated from external noise and vibrations - seismic noise can be 10 times stronger than the signal we are trying to measure! This made some scientists sceptical; in particular, the astronomers were afraid that it would take money away from traditional astronomy. But in 1985, the National Science Foundation [NSF, an American agency that funds fundamental research] agreed to a design study on paper. That was the start of the Ligo project, launched by people like Kip Thorne, Rainer Weiss and Ronald Drever [passed away in March 2017].There were similar projects in Germany and Australia, and one Franco-Italian project. The Australian project never got funding; the scope of the German project, in partnership with Glasgow University in Scotland was reduced to one 600-metre-long interferometer (GEO600) in Hanover. It wasn’t sensitive enough but served as a test platform. Finally, the Franco-Italian project was approved in 1993 and became the Virgo instrument, located near Pisa. It observed its first gravitational waves last summer.
How did the Ligo project go?
There were organisational and personality difficulties that were not able to convince the NSF that they could successfully complete the project. That was when I was handed the reins of Ligo in 1994. I had just jointly managed one of the planned experiments in the Superconducting Super Collider. When the American Congress cancelled the whole giant particle accelerator project, I learned that you have to do everything in your power to keep the support of the funding institutions. I also learned how to bring a research and development idea as far as the final instrument. We reviewed the whole design by proposing to spend more money on the initial infrastructure, so as not to have to rebuild it during later upgrades. We announced that it was “possible” to detect gravitational waves from the very first phase. That meant we were not contradicting any physical law, and that the most optimistic theoretical predictions allowed it. However, we were not making any false promises and we asked for funding so that the research and development for the second phase could run in parallel. We said it was “probable” that gravitational waves be detected during this second phase, called Advanced Ligo. These proposals increased the initial cost of the project by 100 million dollars, but it ensured us the support of the NSF. In total, Ligo has cost about 1.2 billion dollars since 1994, including salaries (about half), instruments and infrastructure. It paid off, because Advanced Ligo detected gravitational waves.
This exploit is not the work of just a handful of scientists. It’s an international collaboration. How did it come about?
In 1997, I created the Ligo Scientific Collaboration (LSC). There were a little over 75 people, including many from Caltech and the Massachusetts Institute of Technology (MIT), the two institutions that carried Ligo at the start. Today we are about 1,000. The collaboration was international from the start, as we need the most brilliant experimenters to attack this problem creatively. My colleagues at Caltech and MIT were a little possessive. I had to convince them to open up the collaboration in a very different way from cooperation in particle physics, where groups that want to join have to provide sufficient technical facilities, instruments or analytical abilities to take part in the project. Like a dowry. We never asked for anything. Whoever had the required personal qualifications could join Ligo, even if it was an isolated professor of physics at a university. This also included foreign scientists; the Australians, whose project hadn’t been accepted by their government, the Germans and Scots from GEO600. Today, the collaboration is very international; however, with 1,000 people and a democratic operation, I believe it has lost in efficiency.
Why do you believe that such collaboration should not be democratic?
It needs to be horizontal, but it also needs leadership that takes responsibilities. Building a large detector is like building a bridge; with a hierarchical organisation, the origin of problems is identified, and these difficulties are moved up to the person who is able to make the decisions. Scientists are more used to working in a horizontal, university environment, which ensures liberty and independence. The two are needed: the part that looks after the detectors, that we call Ligo Laboratory, operates hierarchically, whereas the more scientific part, LSC, is more horizontal; all colleagues have the same status, there is governance with elections to appoint the spokespersons and the group management.
What is the relationship between Ligo and Virgo?
It was a strange mixture of collaboration and competition. Ligo won the race to the first detection, and the competition aspect has disappeared. I believe that the flexible design in stages and open collaboration from the start were the main reasons we got ahead of Virgo, which remained for a long time a uniquely Franco-Italian collaboration and which ran into difficulties perfecting its interferometer. But from the start, we decided to share our data, confirm and compare observations. We are also interdependent: the different orientations of the detectors mean we have access to the polaristion (*) of gravitational waves, which is important information. The orientation is definitively decided on construction. We decided to build the two Ligo detectors with the same orientation, and rely on Virgo to get the polarisation. This third interferometer is crucial for doing triangulation and identifying more accurately the region of the sky from which the gravitational waves emanate.
During the twenty years from your arrival in the project to the first detection of gravitational waves, did you have any moments of doubt?
I oscillated between doubt and confidence in our success. Personally, I have always been an optimist, otherwise I wouldn’t have taken on the project. Compared to experiments like the quest for the Higgs boson at the LHC in Cern, we had far fewer theoretical predictions about what we could detect. We had almost no predictions about the collisions of black holes, only the theoretical concept. As for neutron stars, we knew a couple of binary systems detected by radiotelescopes in our galaxy. But we had to extrapolate to the whole of our galaxy, and then to all galaxies in the sensitive area of the detector. That left a lot of uncertainty. However, I knew that whatever happened, we could still improve the detectors and increase the chances of detection. So, I was full of hope in the long term.
How did you feel on 14th September 2015 when you saw the first signal from the collision of two black holes?
I didn’t cry “eureka”! I was very cautious, as the signal had arrived just a few days after the Advanced Ligo was put into service. I thought it may have been a coincidence or that someone had unknowingly injected false data simulating an event in our IT system. But, during the month that followed, no similar signal was observed, excluding coincidence. Also, we could retrace the data back to the detectors and check that there was no intrusion. In mid-October, we knew the event was real. What to do? By experience, I did not favour publishing the result before we saw other events. I remembered the announcement of the detection of magnetic monopoles by the Spanish scientist Blas Cabrera in 1982, based on just one signal. It turned out to be false. Of course, real discoveries have been made on a single event, like the omega minus particle, in 1964, at the national American Brookhaven laboratory. It was a stroke of luck. But I allowed myself to be convinced by my colleagues. So, we worked very hard to write the article, as we believed that it would become a “classic”, and that it therefore had to be accessible to the layman. During the period preceding the official announcement, we detected a second event and my doubts were completely gone.
What will happen now?
Ligo was stopped last summer for a year and a half in order to perfect the interferometers. We plan to increase the sensitivity by 25 to 50%, to collect data faster and be sensitive to further-away events, i.e. further back in the history of the universe. Then, we will be collecting data of interest to cosmologists (**), and not just astrophysicists. It will take time, but I believe that within two years, it will be a perfectly well-established field. Until then, we already need to start thinking about the next generation of detectors.
(*) The polarisation of a wave describes the direction in which it vibrates.
(**) Cosmologists study the universe in its entirety, whereas astrophysicists look into the physical phenomena that happen in space.
ALREADY MORE THAN FIVE EVENTS IDENTIFIED
The 14 September 2015 and the 17 August 2017 are dates that will probably mark the history of physics, respectively for the observation of the first collision of two black holes and that of two neutron stars thanks to gravitational waves. However, between these two dates, the interferometers detected other collisions of black holes. Three of these signals have been officially validated. Another, captured in October 2015, has not been approved; the probability that it was not a collision of black holes was too high (about 13%, compared to less than 1 chance in a million for the detection on 14 September 2015). When the performances of Ligo and Virgo are optimal, similar cosmic impacts could be seen up to once a day.
> INTERVIEWEE
Barry Barish
Physicist
1936 ▪ Barry Barish was born in Omaha, Nebraska.
1962 ▪ He graduated with a PhD in particle physics from Berkeley University.
1991 ▪ He was appointed professor at the California Institute of Technology (Caltech).
1994 ▪ He became the head of the Ligo project, aimed at detecting gravitational waves.
2002 ▪ The Ligo interferometers started their first phase of operation.
2010 ▪ The first phase of observation ended without detection. Start of the installation of the Advanced Ligo.
2011 ▪ Barry Barish became President of the American Society of Physics.
2015 ▪ On 14 September, Ligo detected the gravitational waves emitted by the collision of two black holes.
2017 ▪ On 17 August, Ligo and Virgo observed the gravitational waves resulting from the collision of two neutron stars.
Kommentare