“Editing the Genome - a Major Revolution in Science”
- Interview with David Liu, Scientist
- 17 oct. 2018
- 10 min de lecture
American scientist, Dr. David Liu, is considered one of today’s most brilliant researchers. His lab’s genome editing technology can be used to correct pathogenic point mutations, or single-letter changes, in DNA. This powerful tool can, in theory, treat diseases that are caused by single-letter DNA changes and potentially provide improvements to agronomic techniques.
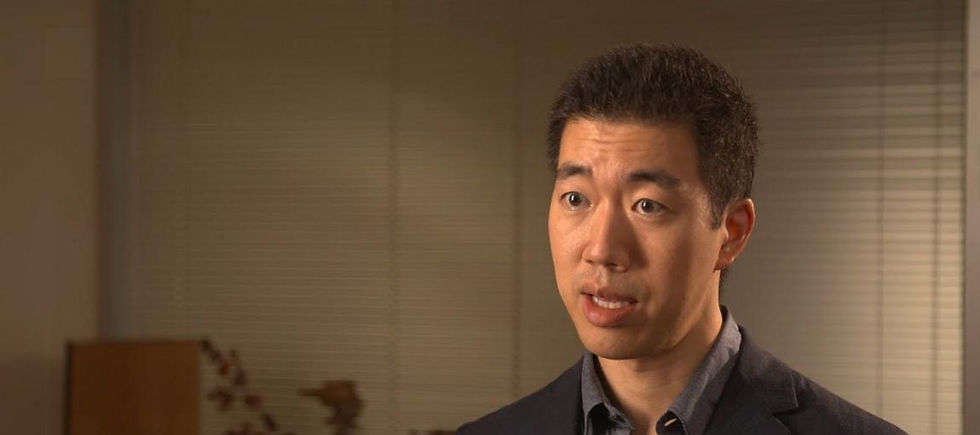
“The Gene Corrector” -- that is the nickname the journal Nature gave Dr. Liu, ranking him among the ten most influential scientists of 2017. A chemist by training, Dr. David Liu has distinguished himself through his genetics research. His work goes further than the CRISPR-Cas9 method, used to modify genome sequences simply, selectively and effectively. Dr. David Liu has developed a technique that can modify a single DNA base pair. This accomplishment has opened the door to potential treatments for diseases caused by such unique errors in the human genetic code. As a member of the Broad Institute at the Massachusetts Institute of Technology and Harvard University, founder of several start-ups, he is seen as one of the most brilliant and prolific biochemists of his generation. He has written numerous major articles, and since this interview, has published four new ones in the promising field of gene editing.
La Recherche • In 2012, CRISPR-Cas9 was invented, a gene editing technique rapidly qualified as revolutionary. What exactly is it?
David Liu • It’s a cheap, effective, and simple technique that uses a bacterially derived enzyme (Cas9) and strands of RNA (*), to manipulate specific sequences of DNA inside a cell. It has been successfully applied in plants, rodents, monkeys and even human embryos. This is one of the most vast and rapid revolutions in the field of the life sciences that I have ever known. It has already profoundly affected basic biology studies, both in clinical research and therapeutic applications.
What could they be?
Firstly, through the use of CRISPR-Cas9, scientists can introduce genetic mutations suspected of causing diseases into cultured cells or guinea pigs, to study their effects and develop a treatment. But above all, they can use gene editing tools to correct or suppress the same mutations wherever they are known to cause illnesses that are currently untreatable; some types of blindness, deafness, epilepsy, neurological and metabolic disorders. Furthermore, in oncology, because being able to correct 100% of tumour cells caused by a genetic defect and managing to stop their proliferation would be extremely challenging, scientists are instead trying other approaches. For example, we can genetically modify immune cells (T lymphocytes) so that they attack cancer cells. Finally, a similar idea is being explored against the AIDS virus (HIV), by making the immune cells insensitive to its assault. The company Sangamo Therapeutics, and other teams, are currently testing this solution in patients.
If CRISPR is so revolutionary, what does the specific technique you developed add?
Of the 64,000 genetic variants potentially associated with diseases (such as sickle cell anaemia, cystic fibrosis, beta thalassemia), two thirds are point mutations. In other words, in the long sequence of pairs of bases or nucleotides (A-T or G-C) making up DNA’s double helix, just a single one of them is responsible for the illness. Methods such as CRISPR have difficulty correcting these errors efficiently and cleanly. In fact, CRISPR (like other gene editing techniques such as TALEN or ZFN), operate like “molecular scissors”; these tools select the DNA at a specific point to remove an unwanted gene, for example. Then, it is up to the cell itself to stick the DNA ribbon back together. Sometimes, this process randomly causes larger and unwanted genetic insertions or deletions, rearrangements, or translocations. Our technique, known as “base editing”,takes a different approach to gene editing than CRISPR. Instead of “scissors”, base editors instead are like pencils. The letters in a pair of bases, A-T for example, are directly transformed into another other pair, G-C. This conversion can directly reverse a mutation that causes a disease. Because we are not cutting the DNA, we are not introducing unwanted elements into it, limiting the undesired changes to the genome . The intervention in the genome is cleaner, as we and others have repeatedly verified in studies.
Concretely, how does this method work?
A base editor is a protein that does not exist in nature. It is made up of components borrowed from nature, but also parts modified in the laboratory. It uses the same biomolecular mechanism as CRISPR-Cas9 to target the desired region of the genome, but it cannot cut the DNA. Rather, it induces a rearrangement of the atoms present, leading to a reconfiguration, a “rewriting” of the pairs of nucleotides. This way of acting directly on the target base pair opens new possibilities for genome editing and also enables some new applications.
Can you give us an example of these new applications?
Of course; we can design systems that describe the internal processes used when a cell reacts to an external stimulus. This is a challenge today. It’s a little like reproducing in the cell the famous “black box” used by commercial aircraft, which records all the events concerning it. We have just developed such a biomolecular system called Camera: when a cell fitted with it is subjected to an antibiotic, to light or to a viral infection, it triggers an editing mechanism in that cell’s genome. Thanks to today’s very powerful gene sequencing possibilities, we can use our system to read the historical sequence of reactions triggered by the cell in the DNA. While the first version, Camera1, used CRISPR-Cas9 tools on bacteria, the second, Camera2, stands on a base editor and can be used to study how human cells operate internally. Two other systems, called Detectr and Sherlock, designed respectively by Jennifer Doudna and Feng Zhang [two of the three discoverers of CRISPR-Cas9], were developed to detect the abnormal presence of foreign DNA, such as a virus in a patient’s blood for example.
Is your base editor, which acts on the components of the DNA, the ultimate solution?
There will never be one “ultimate solution” for problems as complicated and diverse as manipulating the genome. To claim as much would be to presume there will be no more progress made in science. I have seen so many stunning advances recently that I would not bet against scientists’ ability to make other advances. It is true that, if the intention is to modify a pair of nucleotides, the base editor has the unique ability to do so. However, this field of research is so vast that, by using other protein elements, genetic engineering tools, other functions will likely be developed. I believe the field will always move towards finding therapeutic solutions, or to enhance productivity, resistance or other plant characteristics, if we are talking about agronomics. This is one of the first fields where these techniques will be applied. What is interesting is that it is basically the same approach as farmers have used for decades when they cross varieties of the same plant, to improve them naturally by a transfer of genes. Gene editing techniques enable similar interventions but much more efficiently than traditional breeding, so much so that the plants treated with these tools would probably not be considered or regulated as genetically modified organisms (see here).
Coming back to the promising progress in these gene editing techniques, are there limitations? Is the accuracy guaranteed?
Accuracy is a significant challenge, and today nobody knows the degree to which “off-target effects” need to be absent before these technologies can be applied in therapies. It is probable that the answer to this question will depend on the tool and on the application: off-target base editing, for example, may be less consequential than off-target insertion, deletion, or translocation of DNA. And if there is no other treatment for a fatal and imminent disease, off-target effects may turn out to be a minor issue in comparison to other options. That being said, I am very optimistic about the possibilities of using several independent gene editing strategies in synergy to reduce the risk of these effects to an acceptable level.
Another issue sometimes highlighted is the possible propensity of these techniques to trigger an immune response in the host, once the vectors transporting these genetic tools are injected…
All clinicians are aware of this issue. It is unlikely to be so important, as many of the proteins, such as CRISPR-Cas9 exist in bacteria that live in our gut, or on our skin - in the microbiome. There is possibly a pre-existing immunity to these proteins, although they will still work, as described above. One of the most important aspects of gene editing techniques is that they will only be administered to the patient once. I am not too worried about immunogenecity, especially since other immunogenic drugs are already used successful to benefit patients. One potential solution is to temporarily add an immunosuppressant treatment to the therapy.
As these technologies are described as easy to use, what is the risk of them being misused for nefarious purposes? In 2016, James Clapper, then director of United States National Intelligence, even included them in the list of “weapons of mass destruction”.
I do not agree with him. First, if your evil ambition is to harm as many people as possible, there are far more effective means to achieve such a goal than editing their genomes. Yes, these technologies are easy to use, but in the laboratory, for experienced people - not in a bathroom, with just a syringe - which is itself dangerous to manipulate.
This raises the question of clinical trials in humans and not just on cells in petri dishes, embryos or guinea pigs. Where are we on this?
Initial tests are underway in China; others are about to start in the United States. That does not mean that these techniques are ready for widespread use in hospitals; I would hope this to be the case in five to ten years. First though, many questions need to be answered: which genetic targets give the best results with the fewest negative effects? What are the risks associated with the vectors delivering these genetic tools, i.e. viruses? Do long-term tests in animal models illustrate the safety of these methods?
Given the stakes, will all these steps be followed everywhere in the world? China is often pointed out as a country where this type of work is poorly regulated.
On the contrary, I believe that the Chinese scientists are very respectful of regulations, as they want to avoid any incidents. Nobody anywhere wants patients treated with these new techniques to suffer from side effects. The history of gene therapy at the end of the 1990s with deaths in the first clinical trials, then a gap of two decades before they started up again, is vivid in the minds of genome editing scientists. Our field has undeniably benefited from better understanding and scientific developments over the past two decades, both in terms of editing agents, and in terms of delivery techniques.
Would it not be better to think of these issues, especially the ethical ones, before we rush into clinical trials? Some even believe that ethical decisions should be removed from the scientists, to the point of demanding a moratorium…
I believe that all scientists, and not just those involved in gene editing, need to ponder the ethical issues of their field and, most importantly, to communicate them to the public, in scientific journals, to the government, regulatory bodies, so that the best decisions can be made collectively. I do not believe that a complete worldwide moratorium on gene editing is realistic. Frankly, I think that the possible benefits of these technologies are so great that such a radical act would not be beneficial to our societies.
What about a moratorium just on genetic modifications to human germ cells?
A vast debate that leads to the issue of eugenics, transhumanism, etc. Although there is no formal moratorium in the United States today, laboratories are usually reluctant to make genetic modifications to human germ cells. Elsewhere, like in China, where embryos have already been modified, the rules are not as strict. The justification put forward for the authorisation is that the embryos were supposedly not viable. This is true in some cases. In other articles however, this is not clearly specified. I believe it is important to take the time to reflect on the conditions in which genetically editing germ cells is reasonable. That is also why my group is not active in this field. However, common sense tells me that, once again, the possible benefits of taking this step forward (such as preventing serious genetic disease), are too great to ignore. Obviously, huge ethical questions are raised, such as forcing on a future person (the embryo) operations they were unable to consent to. But we don’t ask babies their permission to treat them either; we do so in their best interest. We need to avoid polarising the debate too much.
Some people accuse geneticists of “rewriting the code of life”, of “controlling nature” using these techniques or of “playing God”. These are metaphors widely used by journalists. What do you think of it?
Seeing gene editing techniques as “playing God” suggests delusions of grandeur. Using penicillin to counter bacterial infections that could kill millions of people is no less profound than modifying a mutated gene that causes a serious disease. If these metaphors point to the fact that we are doing research that has a potential impact on the good of humanity, then I accept them.
(*) RNA is ribonucleic acid, a biological molecule that carries genetic information to make a protein, but which may also be non-coding and serve as a guide for proteins, like Cas9, which acts on the DNA.
REPAIRING HEARING LOSS About half of cases of deafness are caused by genetics. Currently, the treatment options are limited, as the loss of hearing caused by a mutation on a gene. David Liu and his coworkers were one of the first teams to use CRISPR-Cas9 to selectively edit a gene that resulted in the partial rescue of an animal model of a human genetic disease. By guiding the enzyme Cas9 to the mutated gene to neutralise it, he managed to reverse hearing loss in mice. “The treatment avoided about 15 dB of hearing impairment: a significant preservation”, explains the scientist, for whom these results are not yet a blank check for CRISPR-Cas9 tests on human deafness, but make it possible to conduct studies in larger animal models. Another concern is targeting a gene whose mutation is often one-off (such as in this form of deafness, which depends on a single irregular DNA base), which may have side effects on the rest of the genome. The solution? The correction was performed in vivo in mice, on cells that had completed differentiation and were no longer dividing. Result: the cascades of molecular signals reactivated, preserving the critical hair cells in the inner ear that otherwise die from the genetic deafness disease (part of the inner ear).
> INTERVIEWEE
David Liu
American scientist, Dr. David Liu, is considered one of today’s most brilliant researchers. His lab’s genome editing technology can be used to correct pathogenic point mutations, or single-letter changes, in DNA. This powerful tool can, in theory, treat diseases that are caused by single-letter DNA changes and potentially provide improvements to agronomic techniques.
1973 ▪ David Liu was born in Riverside, California, to parents of Chinese origin. 1994 ▪ He graduated with a degree in chemistry from Harvard, under the guidance of the Nobel Chemistry prize winner Elias J. Corey. 1999 ▪ He received his doctorate and became assistant professor at Harvard. 2005 ▪ He became a full professor at Harvard and was appointed to the Howard Hughes Medical Institute. 2013 ▪ He jointly founded Editas Medicine, a medical company exploring the potential of CRISPR-Cas9. 2016 ▪ He became director of the Chemical Biology and Therapeutic Sciences Program, at the Broad Institute (Massachusetts Institute of Technology and Harvard). 2017 ▪ He was nominated by the journal Nature as one of the ten most influential scientists of the year 2017. 2018 ▪ In March, he jointly founded Pairwise Plants, a company backed by Monsanto, using CRISPR-Cas9 to enhance plants and, in May, Beam Therapeutics, using the therapeutic potential of “base editors”.
Comments