“Several Models Exist to Describe the Primordial Universe”
- Interview with Robert Brandenberger
- 30 oct. 2017
- 8 min de lecture
Shaking the world of cosmology is a debate between the proponents of cosmic inflation, in the large majority, and a handful of physicists thinking outside the box and highlighting unique solutions for describing the primordial Universe. For the moment, there is insufficient data to plump for either side, believes cosmologist Robert Brandenberger. Interview conducted by Philippe Pajot

La Recherche What is inflation?
Robert Brandenberger Inflation is a model we use to describe the primordial Universe, just after the Big Bang. It is the rapid (well under a few billionths of a billionth of a second), and considerable (exponential) expansion of the Universe. Following this inflationary phase, the Universe continued to expand, but at a much slower rate. And it is still expanding to this day. Although it implies that the distances between points in close proximity grow at a speed faster than light, this expansion does not in fact contradict the absolute law of special relativity. This states that matter or information cannot travel faster than the speed of light. But what is expanding is not the matter, but the space containing it, its geometry so to speak. In technical terms, it is the metric (*) which is expanding. This also applies to the continued expansion today.
Where does the idea of a phase of rapid expansion of the Universe come from?
In the 1970s, Soviet physicist Alexei Starobinsky, from the Landau Institute of Theoretical Physics near Moscow, proposed the exponential expansion of space (1). In this initial work, the expansion resulted from changes to Einstein’s equations of general relativity describing gravitation, to take account of the quantum effects that must have been present in the primordial Universe. Then, Alan Guth at the Massachusetts Institute of Technology (MIT), proposed an exponentially expanding model of the primordial Universe based on scalar field theory (**). This model did not work correctly though and it was the Russian Andrei Linde, today at Stanford University, and the American Paul Steinhardt at Princeton, who, at the start of the 1980s, proposed tangible examples of inflation (2).
Were these models well received?
The enthusiasm was instant. Two years after prepublication, Alan Guth, then studying for his post-doctorate, was offered a professorship at MIT. Success was so fast because the inflation model solved several issues with the standard model of cosmology.
Which problems did inflation solve?
There were three. First, the horizon problem. Observations of the cosmic microwave background, the backdrop to the whole Universe observed accurately by the Planck satellite, shows that at the time this radiation was emitted, 380 000 years after the Big Bang, the large-scale Universe was uniform and isotropic (i.e. the Universe looks the same regardless of the direction from which it is observed). There is no reason the Universe should have become isotropic - for example that the temperatures evened out by thermalisation - if distant regions in the Universe could not exchange information. Information exchange only happens at best at the speed of light and these regions were too distant to have been in contact so early after the Big Bang. The inflation paradigm solves this incompatibility by supposing that the whole Universe was initially contained in a very small space, which could have thermalised and given rise to a uniform, isotropic Universe. Then, the expansion phase inflated the Universe exponentially, moving different parts far from each other and resulting in the Universe we see today (see inset p.40).
Did the standard model of cosmology have other issues?
Cosmologists were also confronted with the enigma of the flatness of the Universe. Space-time is flat - its geometric curvature is close to zero. How could this observation be explained when, from a physical point of view, this would require extremely fine adjustment of cosmological parameters? With exponential expansion, whatever the value of the initial curvature, after the inflation phase, it becomes as small as we want (Fig. 1). As close to zero as necessary. Finally, the problem of entropy: how is it that the entropy in today’s Universe is so large, whereas the initial entropy of the Universe (Planck’s entropy) is very small. Once again, this problem is solved by inflation.
Fig. 1
The Flatness of the Universe
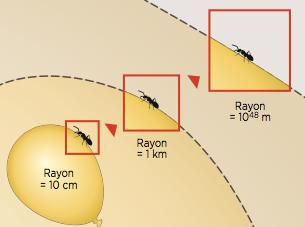
Placed on the surface of a wildly inflating balloon, an ant will no longer notice the curvature. Similarly, when inflation expands the Universe hugely, we see it as flat, whatever its initial curvature: inflation also explains why the Universe appears flat.
Despite its success, inflation is encountering increasing opposition. Why?
Firstly, this opposition is relative: most cosmologists accept the idea of inflation, even if some details remain to be specified. Recently, the debate centred around an article (3) by cosmologist Paul Steinhardt who, as I mentioned, is one of the original founders of the inflationary models, alongside Alan Guth and Andrei Linde. Today, he is strongly opposed to inflation, claiming that it is only a theory and that it never made predictions. He is proposing a concurrent, “ekpyrotic” model, [from the Greek ekpyrosis, which means “conflagration”, referring to the Stoics’ cyclical vision of the Universe, editor’s note], according to which the Universe underwent cyclical phases; the Big Bang is replaced by a sort of bounce from a previous state of the Universe in contraction (see p. 46). His scathing criticism of inflation received a sharp response from around thirty physicists and cosmologists who hold true to this model, including Alexei Starobinsky, Alan Guth and Andrei Linde, but also from Stephen Hawking, Steven Weinberg and Edward Witten, to name but a few.
And what is your position in this debate?
I would first like to say, that while there are several models describing the primordial Universe, inflation is the only one with verified predictions. These predictions are those observed today using instruments such as the Planck satellite, which studies cosmic background radiation. They have drawn up a map of temperatures in space and how they are fluctuating. While this radiation is at an average temperature of 2.7 Kelvins (about -270.4°C), it does fluctuate slightly between different regions in the sky. From these fluctuations, a power spectrum (***) of cosmological fluctuations can be built, which is a sort of signature of the state of the Universe at this far-off time. The simplest inflation model, proposed by Starobinsky, predicts that this spectrum is almost scale-independent, i.e. that the fluctuations do not depend on the scale at which they are observed. In this model, the parameter is equal to 0.96. If there was scale invariance, this value would be equal to 1. And this is the value that was measured by the Planck satellite well after the work on inflation. So in this sense, we can say that the inflation models did indeed make predictions. I do not agree with Paul Steinhardt when he claims that inflation is non-scientific.
So you agree with inflation?
Not exactly. I did not sign the article answering Steinhardt’s criticisms. I wasn’t asked to. Two other renowned cosmologists, Viatcheslav Moukhanov and Neil Turok did not sign it either. While inflation has the advantage of having made verified predictions, there are other paradigms for the primordial Universe that also solve the issues with the standard model of cosmology.
What are they?
This is the case for Steinhardt’s ekpyrotic Universe, the “pre-Big-Bang” cosmology of the Italians Maurizio Gasperini and Gabriele Veneziano, and the “matter bounce” paradigm I proposed with Fabio Finelli. However, like inflation, these are not theories - they are missing the physic bases which would give them this status. General relativity is a theory - it has well-established bases (Einstein’s equations) and all the tests used to verify these predictions have been conclusive so far. The equivalent remains to be found to describe the primordial Universe, be it string theory or another field theory that would give a physical foundation to all these models. For the moment, the profound reason behind inflation, whatever generated this extraordinary inflation, is something called an “inflaton” and which remains very hypothetical. Its physical nature is a scalar field, similar to the Higgs field (the one of the boson of the same name). Deeper understanding of its origin - Higgs boson, string theory, supersymmetrical extension of particle physics, exotic theory - escapes us.
Will there be observations that could decide between these models?
To come back to the example of Einstein’s general relativity, not only did the theory explain previously mysterious ancient observations, such as disturbances in the orbit of the planet Mercury, but it also made many other predictions that have all been verified. For inflation, I said that one prediction had been verified (see p. 42). And that’s great, but that’s all.
Now, I believe that future observations will enable us to arbitrate between the various models. One of these observations concerns primordial gravitational waves. Like the recently-detected gravitational waves resulting from the fusion of two black holes, these are regular vibrations in the space-time continuum. Primordial gravitational waves are different in nature however. Quantum fluctuations in an expanding Universe generate them automatically, at the same time as fluctuations in density. All the models of the primordial Universe predict the existence of these waves. Only their spectrum differs according to the model. For inflation, the spectrum is “offset to the red” (longer wavelengths). For other models, the spectrum is “offset to the blue” (shorter wavelengths).
These primordial gravitational waves are undetectable by any means we could imagine. Will we never find a conclusion?
It’s true they seem undetectable directly, as the changes in space-time they engender are minuscule. But they could have left a trace in the cosmic background radiation. Because they can polarise it, i.e. give a preferred orientation to the electrical field of the background.
Is that what the Bicep 2 experiment announced it had detected?
Yes. In 2014, the Bicep 2 experiment declared, with a lot of publicity, that it had confirmed inflation, as the scientists claimed to have detected a trace of primordal gravitational waves in the cosmic background. Not only was it shown that what they saw was in fact contamination by the polarisation of dust from the Milky Way, but if what they had measured was a true cosmological signal, it would not have confirmed inflation but quite the contrary, as the signal announced was offset to the blue, and not the red. There is a lot of propaganda in this realm. A lot of caution is called for.
Is there an untroubled way out of this debate?
Of course. One cannot deny observation. If the data speak and are undeniable, one can only concur. I eagerly await the results of the observation of the polarisation of the cosmic background radiation by the South Pole Telescope, and its new SPT-3G detector which should soon be operational in the Antarctic. This set of data, combined with those from the ACT, the millimetric telescope in the Chilean Atacama Desert, should clarify things within a few years. Patience will bring its reward.
(1) A. A. Starobinsky, JETP, 30, 682, 1979.
(2) A. D. Linde, Phys. Lett. B, 108, 389, 1982 ; A. Albrecht and P. J. Steinhardt, Phys. Rev. Lett., 48, 1220, 1982.
(3) A. Ijjas et al., Sci. Am., 316, 32, 2017.
(*) A metric, or metric tensor, is a mathematical object used to define and calculate distances and angles in a space or in space-time.
(**) A scalar field is a quantity that associates a number (a scalar) to each point in space-time.
(***) The power spectrum of fluctuations characterises the intensity of fluctuations according to the scale on which they are produced.
MORE THAN 1026
this is the expansion factor of the Universe during inflation.
CONTEXT
The primordial Universe evokes a sudden and rapid expansion of the space-time continuum: inflation. This exponential growth, imagined in the 1980s, defies imagination yet resolves one of the most pressing issues in cosmology. Ad hoc solution or theory? Opinion is divided.
THE HORIZON PROBLEM
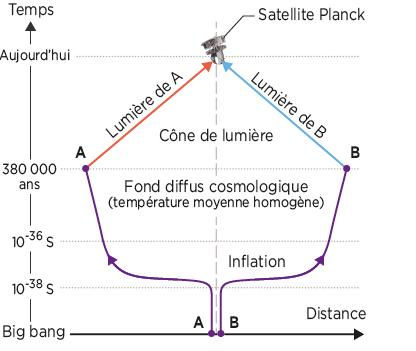
As far back as we can see in the Universe, into the past therefore, we observe the same properties on average. In particular, the trace of light emitted 380 000 years after the Big Bang, the cosmic background radiation, appears very evenly distributed: regions A and B are at almost the same temperature. Between the Big Bang and the emission of the background radiation, no signal could have been exchanged between A and B. The inflation solution: regions A and B, and all other regions in the Universe, were originally very close together so that their temperatures were homogenised before inflation separated them.
Comments