Fighting Alzheimer’s with Gene Therapy
- Nathalie Cartier
- 28 oct. 2017
- 10 min de lecture
Disturbance in the brain’s cholesterol metabolism is one of the factors responsible for Alzheimer’s disease. The gene therapy that we have developed inserts a specific gene into the neurons of the hippocampus and can correct the part of the brain involved in memory.
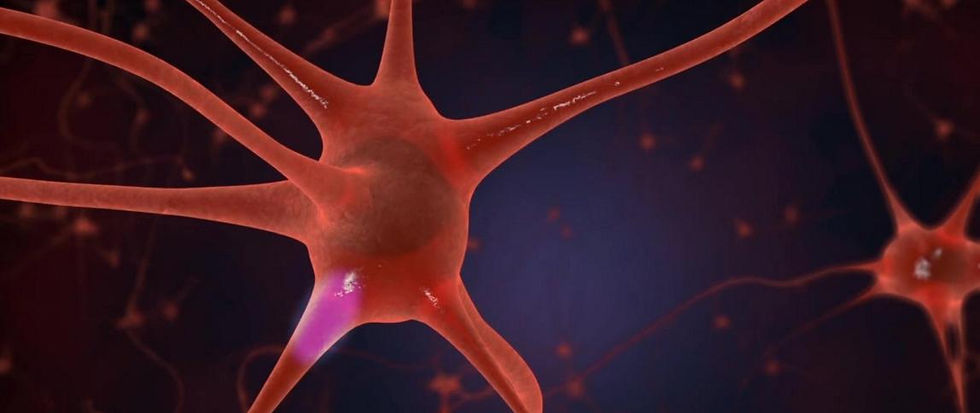
Incurable, Alzheimer’s disease is a neurodegenerative illness that leads to the gradual and irreversible loss of cognitive functions, in particular memory. It is therefore a major challenge to society to treat it. According to the Alzheimer’s Association, delaying the onset of the illness by 5 years would reduce the number of cases around the world in 2050 by 50%, and would represent a saving of 470 billion Euros. Alzheimer’s disease is complex and multifactorial. It is characterised by the appearance of two types of lesions in the brain: amyloid plaques and neurofibrillary tangles.
No effective treatment exists to date. We therefore looked at a new therapeutic target: brain cholesterol. A disturbance in the brain’s cholesterol metabolism hinders the activity of neurons and is involved in Alzheimer’s disease. By using gene therapy to restore the brain’s cholesterol cycle, we succeeded in reducing the amyloid plaques and improving the function of the neurons (1). To treat the disease, we first need to understand how it functions.
Toxic Fragments
The first lesion, amyloid plaques, is caused by a protein, APP (amyloid precursor protein). When it is cleaved by beta and gamma secretase enzymes, it gives rise to small fragments called amyloid-beta peptides (or Aß). These toxic fragments accumulate outside the neurons and clump together into amyloid plaques. The neurofibrillary tangles result in excessive additions of phosphate groups on a protein, called tau. In a normal brain, this protein interacts with the neuron microtubules to maintain them and ensure their flexibility. Microtubules are very thin tubes located in the neurons which convey nutrients and other elements essential to the operation of the cell. The interaction of the tau protein with the microtubules depends on the level of addition of phosphate group - its level of phosphorylation. The more phosphorylated it is, the less it interacts with the microtubules. In this case, the tau proteins detach and clump together; the circulation of the elements through the microtubules is then compromised and the neuron gradually deteriorates.
Which lesion occurs first? The amyloid plaques or the intracellular accumulation of abnormally phosphorylated tau proteins? This question remains open. Since the 1980s, the “amyloid cascade” hypothesis suggests the initial role of the amyloid pathology. It could contribute to the neurofibrillary tangles, with the whole leading to the appearance of synaptic defects followed by neuron death. Despite the large number of drugs assessed in clinical trials, the only treatments available today are procognitive agents, i.e. drugs that delay the development of disorders. These symptomatic treatments are not very effective unfortunately, leading the French Health Authority (HAS) to stop reimbursing them in December 2016.
Two therapeutic research strategies have been used for a long time to find the cause of the illness; reducing the amyloid plaques and the clusters of tau protein. For the amyloid plaques, we have tried to directly destroy the Aß peptides using vaccines or anti-Aß antibodies, to inhibit the enzymes responsible for producing these peptides (the beta and gamma secretases), or to promote the hippocampus’s ability to get rid of the plaques by using cell “cleaning” mechanisms, such as autophagy (*). The “anti-tau” strategies consist of eliminating the clumps of tau protein using antibodies or by limiting their phosphorylation.
Both strategies have proved fruitless, despite the budgets spent by major pharmaceutical laboratories on their development (see inset). Recently, the American pharmaceutical company Eli Lilly’s drug, Solanezumab, which acts on the amyloid plaques, was halted after phase 3 trials (**) on 2,100 patients due to its ineffectiveness.
Nevertheless, some reduction in the number of amyloid plaques was observed by imaging in patients treated with anti-amyloid antibodies. The failure of these clinical trials could be due to the fact that the patients were treated at too late a stage in the disease. The appearance of the plaques and tangles probably commences decades before the late cognitive signs reveal the disorder and the death of neurons (2). Biogen claims as much and is attempting to obtain positive results in a phase 3 trial for Aducanumab (anti-Aß antibody) by including patients at an early stage. These failures have led the search for the early factors involved in triggering the disease and which could open up new, more effective treatment avenues.
Three Families of Genes
In this context, numerous studies have been conducted to identify the factors of genetic predisposition. They have led to the discovery of twenty or so genes associated with the illness, grouped into three families: inflammation, cell transport (endocytosis) and lipid metabolism. In the latter family, several fat transporters are involved. Apolipoprotein E (ApoE) has long been known for its link with Alzheimer’s disease. It transports cholesterol in the brain. There are three different versions (three alleles) of the gene encoding ApoE. With age, the ApoE4 allele is the main risk factor in the disease. To have an ApoE4 allele triples the risk of developing the disease; having two multiplies the risk by twelve. On the other hand, the ApoE2 allele is protective.
This link with genes involved in the lipid metabolism demonstrates the well-established, key role (3) of lipids, and more specifically of cholesterol in the development of Alzheimer’s disease. The disease is associated with excess cholesterol in the membrane of brain neurons. This excess promotes cleaving of the amyloid plaque precursor protein by the beta, and then gamma secretase enzymes, and hence the formation of amyloid peptides. Brain cholesterol is synthesized by cells in the central nervous system. The neurons’ need for cholesterol is very high. It is used in the constitution of their membranes which play an essential role in the formation of synapses, neuron exchanges, synaptic transmission and the production of Aß peptides. In fact, the brain contains 25 % of all the cholesterol in our body. However, excess cholesterol disturbs membrane fluidity and as a result, neuron function. The cholesterol content of neurons must be precisely regulated.
This is not an easy task; as cholesterol cannot pass through the blood-brain barrier, it is produced entirely in the brain. To be evacuated, it must be converted into 24-hydroxy-cholesterol, a compound able to cross the barrier and pass through the blood into the liver, where it is eliminated in the bile. The enzyme responsible for this conversion is cholesterol 24-hydroxylase, also known as CYP46A1. This is a key enzyme in the brain’s cholesterol metabolism (4). By eliminating excess cholesterol, CYP46A1 activates the brain’s cholesterol synthesis pathway. It thus renews it and synthesises intermediary compounds that play a major role in neuron function, such as plasticity, the creation of new synapses and synaptic transmission. Interestingly, it has been shown that activating CYP46A1 is an essential element in the stress response of neurons; it is therefore essential for their survival (5).
We therefore put forward the hypothesis that the enzyme CYP46A1 could be an interesting new treatment target for Alzheimer’s disease. To test this hypothesis, we used mice affected by the disease. The idea was to determine if the increased expression of CYP46A1 in regions of the brain particularly affected could improve their clinical and physiological condition. How could the expression of CYP46A1 be effectively targeted, in the right place? To do so, the neurons where CYP46A1 is usually present would need to be specially targeted, i.e. in the hippocampus, the region of the brain responsible for memory. Unfortunately, there are no activator molecules for CYP46A1 that meet these criteria. We therefore decided to import the gene that codes for CYP46A1 directly into these neurons, using a gene therapy tool.
Trojan Horse
The principle of gene therapy is to insert a gene (a fragment of DNA encoding a protein) into certain cells for therapeutic reasons. This process can compensate for a defective gene in a genetic illness, or provide a beneficial protein to the cell (growth factor, enzyme, neurotransmitter, etc.). To deliver the gene therapy to the cells, we use modified viruses as transfer vectors, which act like a “Trojan horse”. The virus is rendered harmless, but retains its ability to insert its genetic material into the cell. Once the gene is integrated, the treatment protein is produced by the cell permanently. So, only one administration is required for long-term effects. Which vector to use?
Adeno-associated viruses (AAV) are small, non-toxic viruses, commonly used for gene therapy in humans, and proven to be effective in treating illnesses of the retina and the liver. They are suitable for targeting genes in the brain, specifically in the neurons, and are stable over time. One administration is sufficient to enable therapeutic expression of the gene over ten years! To test our hypothesis, we developed a gene therapy vector; an AAV to carry the gene coding for CYP46A1. We administered this drug vector to the mice modelling Alzheimer’s disease, directly into the hippocampus (Fig. 1). At the same time, one group of mice received an inactive control vector.
The animals were monitored clinically for several months, using the Morris water maze. A mouse is placed in a circular pool filled with opaque water. A fixed, immerged platform, enabling the rodent to rest and stay out of the water is invisible. The process relies on the animal’s aversion to water, by measuring the time it takes to find the platform and, by extension, its ability to memorise its position using visual and spatial clues.
Subsequently, the biochemical and neuropathological characteristics of the animals were analysed in detail. Result: the mice that received the drug vector all showed clear signs of clinical and biological improvements, regardless of the severity of the disease. Their memory improved, or even became entirely normal, the accumulation of toxic Aß amyloid peptides was reduced and the consequences of tau protein neurofibrillary tangles were corrected. The treatment restored neuronal plasticity and preserved their ability to create synapses. With this study, we proved that gene therapy is effective in reducing the excessive quantity of membrane cholesterol in affected neurons, improving their function.
Hippocampus Atrophy
We then tried to complete these data showing the beneficial effects of the CYP46A1 enzyme on Alzheimer’s disease. We therefore tested to see if suppressing its production in the brain of a normal mouse would have harmful effects, or would even reproduce some of the clinical and physiological aspects of Alzheimer’s disease. This was indeed the case, confirming the key role played by the CYP46A1 enzyme. By injecting the hippocampus of normal mice with an AAV vector carrying a DNA sequence that blocks production of the enzyme, we increased the cholesterol contained in the lipid membrane, the production of toxic Aß peptides and that of the abnormally phosphorylated tau protein. The mice then developed memory issues and atrophy of the hippocampus - characteristics resembling those of Alzheimer’s disease (6)!
All our results confirm the key role played by cholesterol in neuron function, including the genesis and progression of Alzheimer’s disease. They suggest that cholesterol could be an extremely interesting target in treating the disease. As we mentioned earlier, there are no molecules that can be administered to effectively activate CYP46A1 in the brain and induce its beneficial effects. We are therefore working on transposing this gene therapy approach to bring gene therapy to patients’ brains widely and without risk. Success in this stage will be the key to conducting initial clinical trials in humans, and a step towards a lasting solution in the fight against Alzheimer’s disease.
CONTEXT
Faced with the repeated failure of drug treatments for Alzheimer’s disease, scientists are turning to other approaches, such as immunotherapy or gene therapy. According to the World Health Organisation, 35 million people will be affected around the world, a figure which could reach 115 million by 2050.
MUCH EFFORT, FEW RESULTS
In over 400 clinical trials, 244 compounds were tested in the decade 2002-2012. Among those that reached phase 3 (a crucial stage where scientists test drugs in large numbers of patients in comparison to placebos), almost all were rejected. The tests showed negative results, or worse, presented serious side effects. Jeffrey Cummings, the American doctor who conducted this analysis in 2014, concluded that 99.6 % of clinical trials were failures.
Most of the therapeutic strategies tested concerned reducing amyloid plaques and aggregates of the tau protein. They have so far proved fruitless, especially in patients at moderate and severe stages of the illness. Today, the goal is to conduct the tests in patients at very early stages of the disease, or even before the symptoms appear. At the start of the 2017, 28 compounds were evaluated in 42 phase-3 clinical trials (1): 15 of them are agents targeting the amyloid plaques, and 8 are assessed in early-stage patients or symptomless patients but with a genetic predisposition for the illness.
(1) J. Cummings et al., Alzheimer & Dementia, 3, 367, 2017
THE KEY ROLE OF THE MEMBRANE
The membrane is the essential element enabling the neuron to perform its functions of exchange, synaptic transmission, survival, etc. It is comprised of a bilayer of lipid molecules, in which the membrane proteins are embedded. The membrane lipids are phospholipids, cholesterol and glycolipids. There are zones in the membrane that concentrate more cholesterol. This concentration forms rigid platforms, life rafts on the surface of the membrane, preferred areas for the activity of some proteins. These rafts are involved in releasing neurotransmitters and hence in the propagation of nerve signals. The membrane and its lipid rafts are constantly being renewed by the neurons, requiring the synthesis of new cholesterol. This is produced directly in the brain. The cholesterol content determines the level of fluidity of the membrane. Excess cholesterol stiffens the membrane and hinders its operation.
(1) E. Hudry et al., Mol. Ther., 18, 44, 2010 ; M. A. Burlot et al., Hum. Mol. Genet., 24, 5965, 2015.
(2) C. R. Jack Jr and D. M. Holtzman, Neuron, 80, 1347, 2013.
(3) J. E. Vance, Dis. Model. Mech., 5, 746, 2012.
(4) J.-M. Dietschy and S. D. Turley, J. Lipid. Res., 45, 1375, 2004.
(5) M. G. Martin et al., Neurobiol. Aging, 32, 933, 2011.
(6) F. Djelti et al., Brain, 138, 2383, 2015.
(*) Autophagy is the process by which a cell breaks down its own components that have become useless, dysfunctional or toxic, such as aggregated proteins.
(**) Phase 3 in a clinical trial is the step testing the treatment on a large number of patients, in conditions as close as possible to normal conditions of use.) Phase 3 in a clinical trial is the step testing the treatment on a large number of patients, in conditions as close as possible to normal conditions of use.
> AUTHOR
Christina Smolke
Bioengineer
Christina Smolke is the head of the bioengineering lab at Stanford University, where she teaches bioengineering and chemical engineering. She is also the CEO and president of Antheia, a company specialising in synthetic biology, informatics and fermentation technology.
コメント