The Uncertain Future of the Antarctic
- Olivier Gagliardini, Gaël Durand
- 26 oct. 2017
- 9 min de lecture
Olivier Gagliardini and his colleagues used a numerical model to quantify the current health of floating ice-shelves along the Antarctic. Uncertainties remain about its future however. Several scenarios are being envisaged; the Antarctic could contribute to a rise in sea level of up to one metre by 2100.
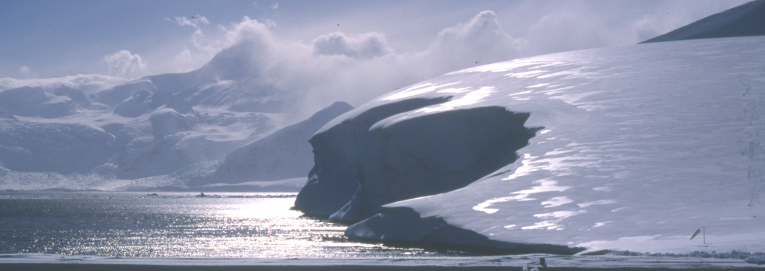
Photo credit: © CNRS Photothèque / ANDRE Marie-Françoise
Between 10th and 12th July, a gigantic piece of ice, slightly smaller than the island of Corsica, broke away from the Larsen C floating ice shelf in the Antarctic Peninsula. This phenomenon has increased concerns about the future of this immense freshwater reserve in a warming world. What influence on its future will this event has(?) in the short, medium and long terms? Scientists are attempting to answer this question. The concern is well-founded; total melting of Antarctica would raise the level of the oceans by about 60 metres! It is a continent of extremes; the lowest recorded temperatures on the surface of the earth (-92°C, measured in the centre of the Eastern Antarctic plateau), the thickest layers of ice (that can reach 4 km), the lowest population density (no permanent inhabitants), and the lowest rainfall, making it the largest desert in the world. On the surface, light snowfalls feed the massive weight of ice that flows very slowly outward on its base, from the centre to the edges. When it reaches the edge and exceeds the grounding line, which separates the fixed part of the ice sheet from the floating part, it floats on the ocean. This is where it starts to change the sea level. Ice is lost through calving (*) of icebergs, usually less spectacular in size that the one that has just occurred at Larsen C.
Understanding the change in sea level therefore means understanding what is known as the Antarctic’s mass balance; the difference between precipitation on the surface of the ice sheet and the ice flow at the grounding line. The precipitation is integrated all over the surface of Antarctica, as the sum of the outflows. It represents huge volumes of ice. Small errors in each one makes it very difficult to estimate this mass balance. However, using satellite measurements of (?) various techniques, we now know that Antarctica is losing about 160 km3 of ice every year and is contributing to 12% of the total rise in sea level of 3.2 millimetres per year currently observed (or 0.4 millimetres per year).
As well as calving magnificent icebergs, the floating ice shelves around the whole Antarctic coast play an important mechanical role in holding back the flow of outlet glaciers further upstream. Like buttressing gothic constructions, these ice shelves form a safety barrier right around Antarctica. If they are weakened by a reduction in size or thickness, it is easy to see how the flow at the grounding line will be increased. And hence the speed of the rise in sea levels. Following a 10% loss in its total surface area, what will become of Larsen C and the ten or so glaciers feeding into it? Surprisingly, this spectacular event will have very few consequences in the short term! This enormous iceberg was already floating in fact. So there will be no rise in sea level. However, by losing 10% of its surface area, the edge of the Larsen C ice shelf has changed from a convex form into a concave shape. Given the context, it is unlikely that the upstream ice flows will be enough to reform the edge and make it convex.
Forthcoming Disintegration
In the medium term, this concave shape suggests that the Larsen C ice shelf may end up like its neighbours, Larsen A and B, which disintegrated respectively in 1995 and 2005, following a similar change in the shape of their fronts. Larsen C will probably undergo the same fate, but it is difficult to predict when. The departure was discrete, but will have major consequences for the neighbourhood!
In the longer term, its disintegration will lead to the disappearance of the forces being exerted on the outlet glaciers; they will accelerate. Consequence: greater flow at the grounding line, and an increased contribution to the rise in sea levels. This was observed in 2002 for the glaciers held back by Larsen B. The glaciers Hektoria, Green and Evans saw their speeds multiplied by 8 when the Larsen B shelf disintegrated (1), leading to an increase in the ice flow evacuated at the grounding line of 2.7 km3 per year to 23.5 km3 per year.
Today, more than a decade after the disappearance of Larsen B, these glaciers are still unstable and continue to lose mass. Should we be worried about the future of Larsen C? In terms of rising sea levels, the maximum cumulated contribution that could be provided by all the glaciers in Larsen C remains low, of the order of two centimetres. But the impending death of Larsen C means we need to look at Antarctica in its entirety, and its immense potential for causing a rise in sea level. What about the other ice shelves around Antarctica? Are they in good shape? Are they able to resist the thrust of the feed glaciers they are holding back? And for how long? In order to answer these questions, scientists are using numerical models.
Increasingly complete and complex, these tools are being used to reconstruct the pas evolution of the polar ice caps, simulate their current condition and predict their future evolution. This is the case of the Elmer/Ice model developed by several laboratories around the world, including ours at the Institute of Geosciences of the Environment (IGE) in Grenoble. To better define the poorly known physical parameters that control flow, these models can now use satellite observations, such as the speed of the ice on the surface or changes in surface elevation. These data are assimilated to estimate the friction of the ice on the bedrock, an important parameter that cannot be observed visually.
Active or Passive Zones
Using these data, we quantified the current health of all the ice shelves floating around Antarctica (2). For each one, we identified so-called “passive” zones, where the ice could be eliminated without causing significant changes upstream. This is the case for the iceberg that has just detached from Larsen C, as it was fully contained in a passive zone. On the other hand, in so-called “active” zones, calving of an iceberg will have immediate consequences reducing the buttressing effect exerted by the ice shelf on the glaciers upstream. Sea level will be changed. On the scale of the Antarctic, on average, this safety barrier represents 13.4% of the surface of floating ice shelves, with differing distributions around the coast. Some glaciers, such as Mertz and Drygalski, have more than half of their floating shelf comprised of passive ice and are thus not very sensitive to changes in shape. For others, these passive zones are almost non-existent, and any change in shape will have serious consequences on the upstream ice flow. This is the case in particular for the ice shelves in the Amundsen and Bellingshausen seas. The modelling we conducted draws up a picture of the current situation, but does not estimate the future contribution of each of these glaciers to the rise in sea level. This work requires taking account of the evolution of the glaciers over time, their change in shape and the movement of the grounding line. It depends on the climate scenarios showing temperature and precipitation changes on the surface of the ice cap in the future, but also on the change in temperature of the oceans surrounding Antarctica. This is a difficult exercise given the multiple uncertainties.
In the last 2013 report from the Intergovernmental Panel on Climate Change (IPCC), Antarctica’s contribution to the rise in sea levels by 2100 represents only about 5 cm in an overall increase of 44 to 74 cm. The IPCC points out however that greater contributions cannot be excluded, in particular linked to accelerating ice flows in Antarctica. Relatively low, it is bound to be a conservative estimate by the community given the difficulty of the exercise and the then state of knowledge. More recent studies (3) show that Antarctica’s contribution could be far greater by 2100 (more than one metre in the worst-case scenarios). Two mechanisms of instability could considerably accelerate the discharge of ice into the ocean.
The first is caused by the topography of the bedrock located under the ice in the grounding zone. To be stable, the grounding line cannot lastingly remain on the downward slopes along which the glacier flows. Many glaciers have their grounding line just at the summit of such slopes, and only a small disturbance is enough to move the line back. This could be a decrease in the ability of the ice shelf to contain the flow of upstream glaciers. This occurs when they lose ice in a zone that is not “passive”, either through iceberg calving or by melting which occurs in the cavities, between the seabed and the ice ceiling on the ice shelf - a phenomenon which increases with the temperature of the ocean. When the resistance of the floating ice shelves decreases, the upstream glaciers accelerate and their grounding line recedes. In some cases, this line is located just before the summit of a relief. If it recedes sufficiently to pass over this summit and start down the slope, it will recede in a self-perpetuating cycle until it reaches a downward slope (in the direction of the flow). As the receding grounding line leads to a reduction in the part of the icecap resting on the bedrock, the sea level will therefore rise. This process, envisaged at the start of the 1970s, has since been confirmed both theoretically and using numerical modelling (Fig. 1).
Threshold Effect
This is the case for the glaciers flowing into the Amundsen Sea. And we have seen that their floating shelves only have a very small passive zone. For the Pine Island Glacier, the current largest contributor to rising sea levels in Antarctica (13% of Antarctica’s total contribution, or 0.056 mm per year), we believe that this instability has already been reached (4). The same would appear to apply to the Thwaites glacier. If this process has indeed begun, a large part of Western Antarctica could gradually disappear, causing a rise in sea level of more than three metres. The likelihood of such a scenario, like the pace of any shrinkage (from the century to several millennia), remains uncertain however.
The second mechanism of instability at work is the sudden collapse of the front of floating ice shelves. In the event significant climate change leads to the repeated occurrenceof surface melting, the resulting water could fracture the ice shelves, exposing ice cliff rising more than 100m above the glacier fronts. These would probably collapse under their own weight. Progressive collapse of the part resting on the bedrock would occur.
In such a scenario, the Antarctic would alone contribute to a rise in sea level of a little over one metre in 2100. As a result, the receding of the polar ice cap would be considerably accelerated and Antarctica would contribute to raising the level of the sea by more than 15 metres in 2500. By then, not only would the western part of Antarctica be entirely gone, but large sectors to the east of the continent would have receded. For such a disaster scenario to occur, the melting of the surface along coastal areas of Antarctica would have to be steady and significant, which is only envisaged for the most pessimistic greenhouse gas emission scenarios.
We are most likely looking at mechanisms with a threshold effect. Any additional disturbance would lead to a snowball effect which could change the topography of an entire continent, and affect all coastal areas on the planet. The consequences on the whole climate system remain largely unknown to date. How long would this system resist the disturbances before these irreversible changes take place? This is a highly debated research issue with no clear answer. One thing is certain: the more significant the changes imposed by humanity on our climate, the greater the chances of seeing Antarctica suddenly recede. As regards the recent calving of Larsen C, this spectacular event should be seen as a warning from the Antarctic continent if nothing is done to limit our propensity to disrupt the climate machine.
Three Methods One Observation
Advance in satellite measurement have gradually enabled the mass balance of the polar ice caps to be determined. Today, three separate methods deliver the same verdict: the Antarctic Peninsula and the sector of the Amundsen and Bellingshausen Seas are losing ice and contributing to rising sea levels. Altimetry, which measures variations in altitude on the surface of the ice cap, has shown that glacier zones closer to the coast in the Amundsen sector are thinning by several metres per year.
Interferometry, which measures the speed of flow on the surface of glaciers and determines the position of the grounding line, revealed that the speed of the glaciers in the Amundsen sector has approximately doubled since the 1970s. Their grounding lines have also receded, for some by more than 30 kilometres between 1992 and 2011. Finally, gravimetry, which determines changes in ice cap mass, shows an acceleration of loss of mass in Western Antarctica over the last fifteen years.
(*) Calving is the loss of fragments of a glacier in the form of icebergs.
(1) E. Rignot et al., Geophysical Research Letters, doi:10.1029/2004GL020697, 2004.
(2) J.J. Fürst et al., Nature Climate Change, doi:10.1038/nclimate2912, 2016.
(3) C. Ritz et al., Nature, 528, 115, 2015; R.M. De Conto et David Pollard, Nature, 531, 591, 2016.
(4) L. Favier et al., Nature Climate Change, doi:10.1038/nclimate2094, 2014.
Context
The Antarctic contributes about 12% to the overall rise in the level of the oceans. Recently, the peninsula lost an iceberg measuring 5,800 square kilometres, giving rise to new concerns about its future. Using modelling, glaciologists are attempting to anticipate the consequences.
14 million km2 is the total surface area of Antarctica.
160 km3 is the volume of ice lost in Antarctica each year.
> For more information
www.ige-grenoble.fr The website of the Institute for Geosciences and Environmental research (IGE).
www.projectmidas.org The MIDAS project, by Swansea University, in the United Kingdom.
elmerice.elmerfem.org The Elmer/Ice model.
http://isskolen.dk/wp/?page_id=9894 Climate Change Explained to Children.
> AUTHORS
Olivier Gagliardini
Glaciologist
Olivier Gagliardini is professor at Grenoble Alpes University and are studying modelling of glacial flows at the Institute of Geosciences of the Environment in Grenoble.
Gaël Durand
Glaciologist
Gaël Durand is CNRS research fellow and are studying modelling of glacial flows at the Institute of Geosciences of the Environment in Grenoble.
Fabien Gillet-Chaulet
Glaciologist
Fabien Gillet-Chaulet is CNRS research fellow and are studying modelling of glacial flows at the Institute of Geosciences of the Environment in Grenoble.
Comments