The Art of Making Clouds
- Barbara Nozières, physical chemist
- 30 mai 2017
- 10 min de lecture
It is no longer enough to use change in state to explain cloud formation. The role of chemical and biological substances must now be added.
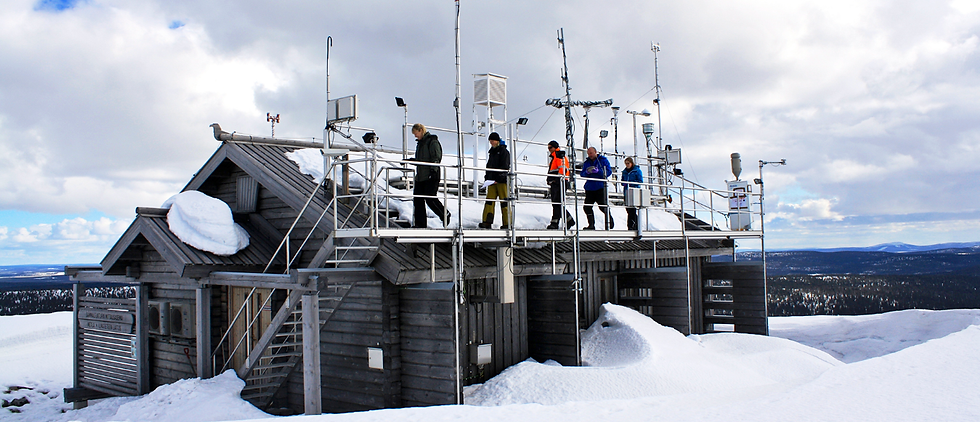
Photo credit A. Frossard
In brief:
Cloud behaviour still escapes modelling.
The geometry and chemical composition of aerosol particles around which water droplets condense must be taken into account.
Substances probably of organic origin, surfactants, play an essential role in lowering the surface tension of the drop.
Say, where do clouds come from? We have all asked this question at one time or another. Conscientiously, we told a wonderful story, of water vapour released from the ground that rises to altitude, meets cold air and condenses into droplets to form a cloud. The thermodynamics of water however are clear: to do so, water would need to be found in concentrations far greater than those observed in the Earth’s atmosphere. The seemingly simple question remains one of the most deep-rooted problems in meteorology. Because no one knows the answer, we are today unable to predict cloud formation, or the number or size of their drops. This in turn makes it difficult to forecast how often they will appear, their opacity or their ability to produce precipitation - rain or snow. Above all, the mystery of cloud formation is one of the main factors of uncertainty in predicting climate change. By reflecting some of the sun’s rays hitting the atmosphere back into space, clouds are the main factor involved in the cooling of the climate. Climate models therefore need to take them into account. Because we are unable to digitally predict the formation of clouds or their properties, these models use data taken from satellite observations. This simplification introduces considerable uncertainty into the climate energy balance, seized upon with delight by global warming sceptics. Several significant advances have been made in understanding this process over the last ten years. Not without effort though: this evolution requires resistance from the meteorological community to be set aside and the admission that cloud formation and properties are influenced not just by physical mechanisms, but also by chemical substances and even biological processes. At first glance, a cloud is a simple thing: a collection of liquid water drops (we shall ignore ice clouds which have an even more complex behaviour). The formation of the liquid cloud seems to come down to purely physical phenomena: when a vast mass of humid air rises to altitude, it cools. If the water vapour content reaches or exceeds saturation vapour pressure (*), the water vapour spontaneously changes into liquid water. The story does not end there however. On a microscopic scale, the formation of water droplets also requires the formation of a frontier between the gas and the liquid, where several processes enter into conflict: the Kelvin effect, which states that water evaporates more easily at the surface of a curve; and surface tension, which holds the water droplets together. These phenomena impose that cloud droplets can only form from pure water vapour when the water vapour pressure exceeds saturation vapour pressure by at least 10%. This degree of over-saturation is not found in the Earth’s atmosphere. And according to these laws of physics, clouds cannot form…
AEROSOLS, KEY PLAYERS
Clouds are here, however, and even cover more than 60% of the surface of the planet! This apparent paradox was resolved by the Swedish meteorologist, Hilding Köhler. In 1936, he demonstrated that the formation of cloud droplets can be explained by water condensing on aerosol particles, tiny solid or liquid particles suspended in the atmosphere (1). The equation he proposes – known today as the Köhler equation – is unanimously accepted by the scientific community. It describes how aerosols facilitate cloud formation in three different ways. Firstly, the aerosols exert a “size effect”. The droplet that forms when water vapour condenses on an aerosol particle from a few tens of nanometres (nm) to a few micrometres (µm) in diameter is already larger than the droplet that can form around a water molecule a few nanometres in size. While the water droplet is larger, the surface of exchange between the liquid and the gas surrounding it is less curved. The Kelvin effect is considerably reduced and the just-condensed water is less likely to evaporate. The second beneficial effect of aerosols on the formation of water droplets comes from Raoult’s law of thermodynamics. This states that the chemical compounds released by the aerosol particles into the droplets significantly reduces the concentration of water vapour around them. This is called the “Raoult aerosol term”, which opposes the Kelvin effect and enables the droplets to grow more quickly. Finally, the third effect is also chemical: some chemical compounds released by aerosols lower the surface tension on the droplets, which also promotes their growth. These compounds are called surfactants (Fig. 1). According to Köhler’s theory therefore, cloud formation is not subject to weather conditions alone (temperature, relative humidity, water vapour content and vertical speed of the air mass). It also depends on the size and chemical composition of the particles serving as condensation nuclei. All these features are of variable importance, depending on the type of aerosol, cloud and weather conditions. In the end though, they determine the number of aerosol particles likely to serve as condensation nuclei. Furthermore, because the quantity of water in the air mass is limited, these characteristics also directly determine the number and size of the drops. The latter are essential in the cloud cycle, especially for precipitation (rain), and for the climate. A cloud formed of large drops will rain more than a cloud comprised of smaller drops. It will also reflect less of the sun’s light into space and will have less of a cooling effect on the climate. Kölher’s theory therefore, teaches us that without aerosols there would be no clouds.
NEW MEASURING DEVICES
For decades however, the role of the chemical composition of condensation nuclei was ignored and even denied by atmosphere physicists. Cloud formation models only took account of their size. This does not just denote a resistance to introducing chemistry into a traditionally physical discipline, it is also the consequence of experiments conducted in the laboratory and in the field: these experiments did not succeed in demonstrating chemistry’s role in water droplet formation. While the effects described by Köhler were real, it was thought that they were negligible. There was another possible explanation for these observations: the instruments usually used to study these phenomena omit the effects of chemical composition as they do not directly measure the corresponding parameters in the Köhler equation (the Raoult term and surface tension). These instruments mainly measure the growth of cloud drops. To do so, they suck in aerosols directly from the air and pass them through a compartment where they are exposed to controlled relative humidity. On leaving this compartment, the number of drops formed or their growth in size is measured. In addition, until recently, there was no analytical technique that provided access to the full chemical composition of these aerosols. These are, by nature, very diverse particles (natural mineral dust carried on the wind, organic particles emitted by plants or human activities, or produced directly in the atmosphere, etc.) and they contain thousands of chemical compounds. For a long time however, chemical analysis of aerosols was limited to just a few types of compounds, as each type required a specific analytical technique. Starting in the 2000’s, the chemical analysis of aerosols was largely facilitated by a new apparatus, the Aerosol Mass Spectrometer, or AMS. It vaporises the chemical compounds in the aerosol particles collected, ionises them and classifies them according to mass. This technique should ultimately enable the majority of the fine particle composition (less than 1 µm in diameter) to be determined. Using the composition of the inorganic salts and organic compounds, it was hoped to establish a link between the chemical composition of aerosols and the Raoult term in cloud formation. Unfortunately, the scientific community became disillusioned… The many missions during which an AMS was brought into the field (on the ground, on boats, planes) could not establish a link between the composition of aerosols and the Raoult term in the Köhler equation. Experiments in the laboratory however on the formation and growth of drops using aerosols of known composition confirmed the influence of some substances, namely inorganic salts, on the Raoult term in the Köhler equation. What could be the reason for this inconsistency? A first explanation is that the role of the chemical composition of the aerosol on the surface tension, the second mysterious parameter in the Köhler equation, had never been taken into account in these studies. By default, the surface tension of pure water was used. This is especially high, as intensified by the connections that water molecules make between each other (hydrogen bonds). The presence of inorganic salts in the drops tends to strengthen these bonds through electrostatic effects, whereas inorganic compounds weaken them. Numerous organic molecules present in aerosols could therefore act as surfactants, but with an effectiveness that varies by several orders of magnitude depending on their molecular structure.
THE PROVEN ROLE OF SURFACTANTS
While analyses of aerosols and fog have long indicated the presence of surfactants in atmospheric particles, the traditional techniques for studying the growth of drops did not highlight their role in cloud formation. Even in the laboratory, introducing known surfactants into aerosols showed no noted effect on the formation of drops. It should be said that these traditional measuring devices have their limits. In particular, they are unable to recreate the very high relative humidity conditions (98-102%) required for drop formation in nature. They can only study these phenomena up to a relative humidity of about 95%. This means that observations need to be extrapolated to guess the full growth of the drops and then, the number and size of drops formed in the atmosphere. To offset this problem, several Californian teams modified these instruments (2). Their goal? To study these phenomena at humidities approaching 100%. In 2016, thanks to these technical improvements, a team led by Kevin Wilson at Lawrence-Berkeley National Laboratory finally demonstrated the role of surfactants in drop growth (3). Here in France at the Lyon Institute for Research into Catalysis and the Environment (IRCELYON), we revealed another important technical limitation of these instruments. It explains their inability to observe the role of surfactants on droplet growth: their measurement time, i.e. the time it takes the drop to pass through the instrument’s humidity chamber as it forms is too short (4). These measurement times are usually between 0 and 20 seconds. Surfactants, however, do not lower the surface tension of drops instantly. To act, they have to migrate the aerosol towards the drop’s surface. Electrostatic forces considerably slow this migration. By measuring the real-time change in surface tension of drops containing these surfactants, we were able to confirm the existence of this slow-down. The “suspended drop” technique was used: a drop of about 1 millimetre in diameter is formed at the end of a syringe; the change in its shape allows the surface tension to be measured in real time. By extrapolating our measurements to small drops, we deduced that for a drop 1 µm in diameter, the surfactant could only have an effect after about one minute, far longer than the twenty or so seconds of observation of traditional instruments. This estimate was recently confirmed by surface tension measurements directly on drops 1 µm in diameter held in levitation on a laser beam. Following these laboratory results, the effect of surfactants in the real atmosphere remains to be demonstrated however. To do so, we need a method to isolate the compounds in the aerosols or in cloud drops sampled from the atmosphere. This is what we did from 2008, making use of a specific property of surfactants: they prefer to lie at the interface between the water and the air. We developed an elaborate protocol using two stages of “extraction”: one aqueous and the other on a solid surface (“Solid Phase Extraction”). Using this method, we were able to find surfactants in very different aerosols, from a wide variety of geographical regions (coastal zones, forests, cities, etc.). We identified them in most atmospheric aerosols, and at sufficient concentrations to affect the growth of cloud drops. Finally, in 2015, we organised a lengthy field campaign at a site devoted to cloud observation in northern Finland. This site frequently lies inside the clouds at an altitude of 600 m. For nine months, we simultaneously observe the clouds passing through the site and analysed the surfactants in the aerosols. The results showed that the frequency of cloud appearance increased considerably at the same time as a parameter characteristic of surfactants – their “critical micellar ability” – indicating their ability to form micelles (*) in the water. These observations are the first direct proof of the role of surfactants in cloud formation in the atmosphere, exactly eighty years after they were predicted by Köhler.
BIOLOGICAL ACTIVITY
The team also recently demonstrated that 41% of the micro-organisms extracted from clouds produce surfactants and are therefore capable of increasing the probability of cloud droplets reforming on the same condensation nucleus. All this work has revealed the limits imposed by instrument techniques on understanding these mechanisms. But above all, they have underlined the importance of chemistry and biology in explaining the formation of clouds. Without them, the Earth’s water cycle would undoubtedly be very different.(5)This study is only the start. Our analysis has also provided clues as to the origin of these surfactants… and it is surprising. All the surfactants we isolated have the characteristics of biosurfactants, i.e. surfactants produced by micro-organisms living on the surface of the Earth. As well as chemistry therefore, it would seem that biological activity on Earth participates actively in the formation and cycle of clouds. We already knew that 98% of the water vapour in the atmosphere is released by vegetation. For about ten years, we have also known that liquid cloud drops contain active and living micro-organisms. The team of Anne-Marie Delort at the Institute of Chemistry in Clermont-Ferrand, believes that clouds could typically contain 100,000 cells per millilitre of water. They modify its chemical composition by consuming trace nutrients and producing waste
(1) H. Köhler, Trans. Faraday Soc., 32, 1152, 1936 (accessible online: http://aerosols.ucsd.edu/classes/A_SIO209_WK2_Kohler.pdf).
(2) B. Nozière, Science, 351, 1396, 2016.
(3) C.R. Ruehl et al., Science, 351, 1447, 2016.
(4) B. Nozière et al., Nat. Commun., 5, 4335, 2014.
(5) M. Vaïtilingom et al., PNAS, 110, 559, 2013.
CLOUDS AND COSMIC RAYS
Do cosmic rays also play a role in cloud formation? Scientists trying to explain climate fluctuations have for a long time flirted with this hypothesis. Cosmic rays are in fact high-energy particles – mainly protons – produced in the cosmos, arriving in the Earth’s atmosphere and colliding with its constituents. Since 2010, CERN’s CLOUD experiment in Geneva has confirmed that these rays are indeed capable of ionising atoms in the atmosphere and causing aerosols to form (1). However, the number of particles formed by this bombardment is negligible compared to the number formed by other processes at work in the atmosphere. Moreover, they are of particularly small sizes (on the order of the nanometre), considerably reducing the chance of them forming cloud drops. The link with cloud formation would seem not to exist. This is also the conclusion reached by the IPCC group of experts in their latest report.
(1) J. Kirkby et al., Nature, 476, 429, 2011.
> AUTHOR
Barbara Nozière
Physical Chemist
Barbara Nozière studies the reactions, physics and chemistry of organic compounds in the atmosphere, from basic aspects to measurements in the field. A CNRS research fellow, she works at the Lyon Institute for Research into Catalysis and the Environment (IRCELYON), where she leads a Franco-American project on cloud formation, the main agents in atmospheric cooling.
Comments